7 Glaciers and Glacial Landforms (An Ontarian Perspective)
Nahgeib Miller
Learning Objectives
After completing this chapter, you should be able to:
- Define the nature of ice.
- Describe and explain how glaciers form.
- Describe and explain how glaciers move.
- Describe the types of glaciers.
- Describe and explain the formation of glacial landforms, including the Oak Ridges Moraine.
7.1 Glaciers
Currently, we are technically at the end of an ice age, with roughly 12% of Earth covered year-round in ice and snow. This permanent covering originates at the poles and stream outward toward the equator or from mountain tops toward the baseline. About 20,000 years ago, approximately 30% of Earth was covered year-round with ice and snow. It is thought that the ice from the two poles touched only once in Earth’s history during the time of the theorized “snowball Earth” about 700 million years ago (Figure 7.1), during the Precambrian.
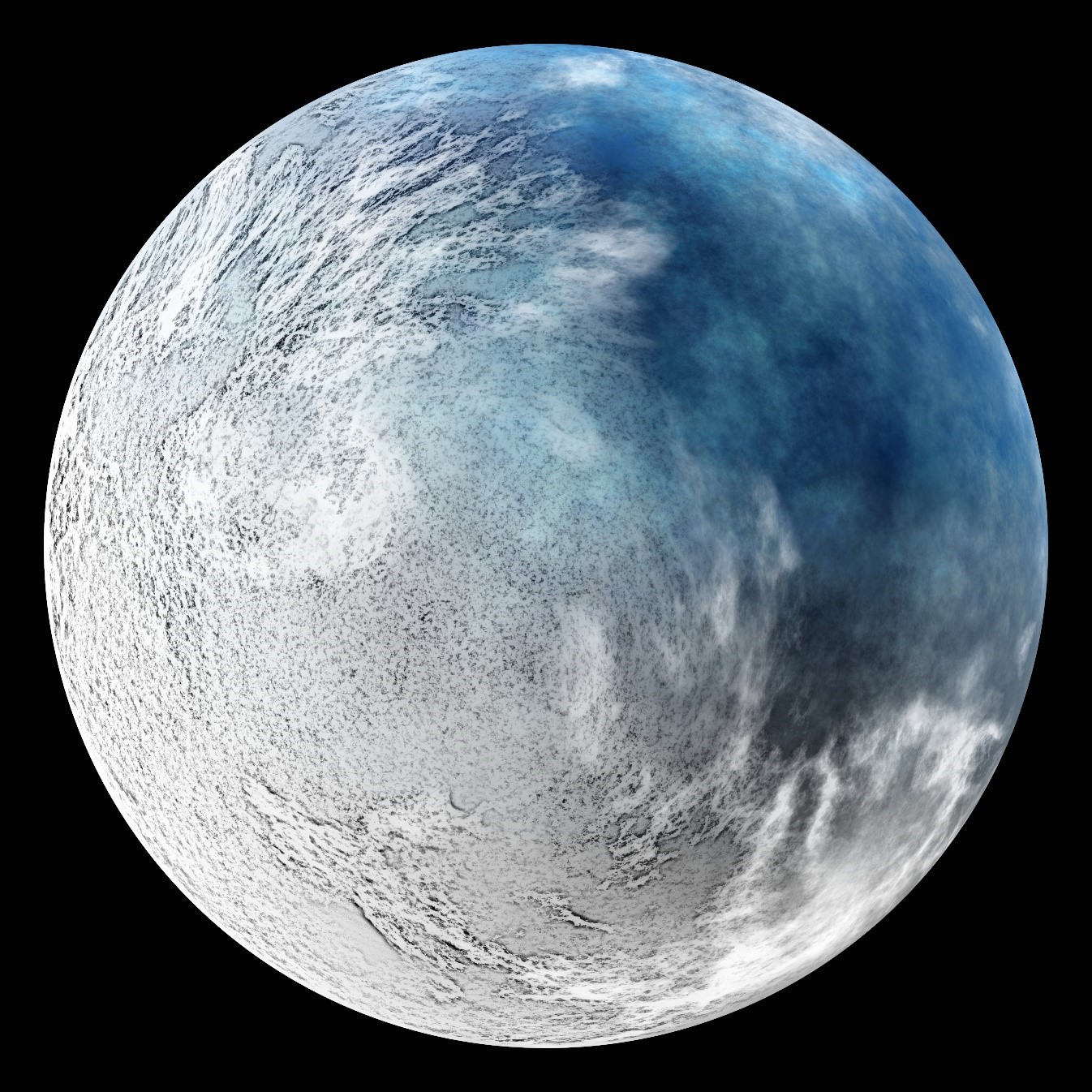
As the ice in a glacier moves, it carves out distinctive features, including steep valleys, and carries large amounts of sediments. At the end of the glacier, pieces of it break off and sediment is carried away and redeposited by meltwater. Therefore, glaciers are a major agent of geomorphology, sculpting the landscape that we see today at Earth’s surface. In fact, much of the morphology of Ontario is a result of glacial action.
The Nature of Ice
For our geologic purposes, a block of naturally occurring ice is a rock — a mass of crystalline grains of the mineral ice. Yes, ice is a mineral! Let us apply the definition of a mineral to see if ice meets the criteria (Table 7.1).
Table 7.1. Ice as a mineral
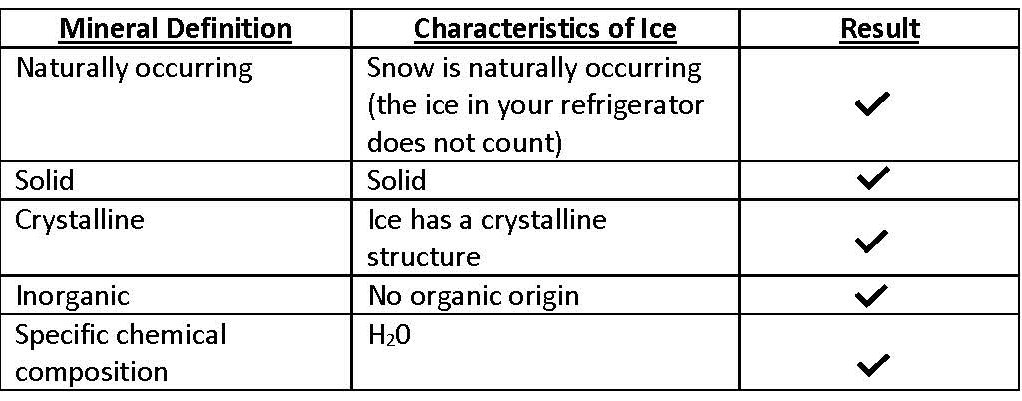
Like most rocks, ice is hard, but ice is less dense than most rocks — in fact, it is less dense than water. This is why ice floats in your drink: water expands upon freezing. Ice can be considered an igneous rock because it originates from a molten liquid (water), and like sediments, snow is deposited in layers at Earth’s surface and can accumulate to great thicknesses. Ice can also be considered as metamorphic rock, because it is transformed by recrystallization under pressure (Figure 7.2). Glacial ice forms from the burial and metamorphism of “sediment” snow as loosely packed snowflakes (each a single crystal of the mineral ice) age and recrystallize into a solid mass. The most unusual characteristic of the rock “ice” is its low melting point. Minerals in igneous, sedimentary, and metamorphic rocks begin to melt at about 700 °C, while the mineral ice starts to melt at 0 °C.
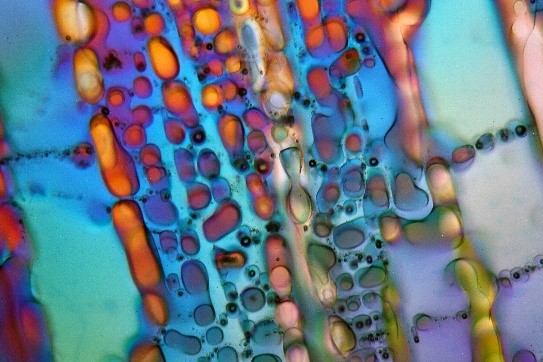
How Glaciers Form
The main ingredients for the formation of a glacier are cold and snow. That is, snow falls in the winter and does not melt in the summer and therefore accumulates in layers. As the layers thicken with subsequent snowfalls, the snow previously deposited begin to be transformed into solid ice through compaction and the removal of air between the grains. Thus the snow crystals are transformed into granular ice called firn, which is about 20% to 30% air. Glacial ice is formed when the air content reaches below 20% (Figure 7.3). When the ice is several tens of metres thick (about 50 m), it begins to flow. The flow is due to the shear weight of the ice inducing plastic flow, like toothpaste out of a tube.
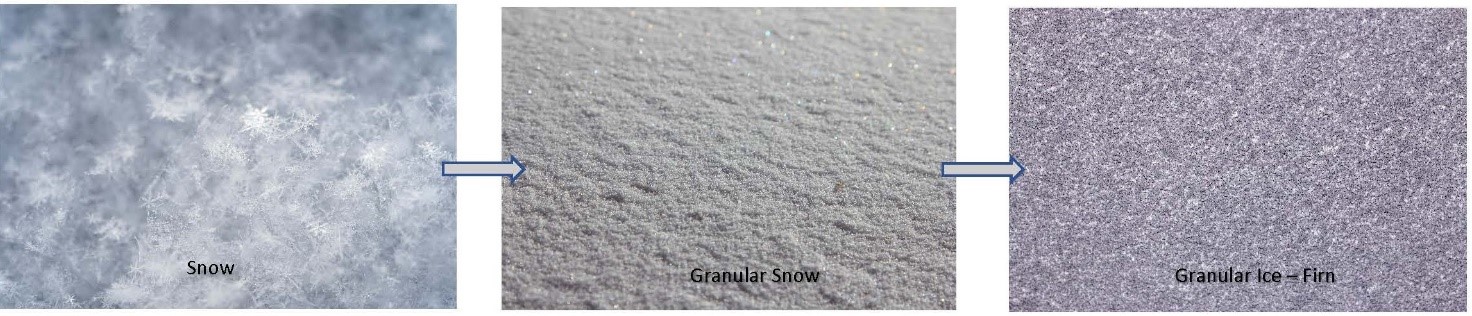
The total amount of snow added to a glacier each year is called its accumulation, and the amount it loses annually is called its ablation. Several processes that are responsible for ablation in a glacier:
- Iceberg calving When a piece of ice breaks off at the front (or end) of a glacier, it is said that it has given birth to a calf, like a cow.
- Melting When the ice melts due to higher temperatures at lower altitudes or due to a warming planet, the glacier loses material.
- Wind erosion Strong winds can dislodge and move particles of ice, leading to loss of material. This is a minor process leading to ablation.
- Sublimation Ice can be transformed from a solid state to a gaseous state without melting.
The majority of a glacier’s ablation is attributed to iceberg calving and melting at its leading edge. When accumulation exceeds ablation as the glacier flows down the valleys or outward from its centre, the leading edge will seem to advance. Conversely, the leading edge will seem to retreat when ablation exceeds accumulation. Imagine you work in a chocolate factory and a conveyor belt carrying treats terminates at the front of the factory where you and your workmates are hastily eating them. If you eat faster than the conveyor belt can deliver the treats, then you must move continuously up the belt, closer to the back of the factory, where the treats originate. Thus, it would seem that the “front” of the belt (where the majority of the ablation is happening) is “migrating” or retreating (Video 7.1).
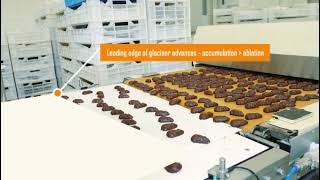
The conveyor belt moves in the direction of glacial flow. Notice that movement is continuous, even in glacial retreat. Click on the image to load the video.
How Glaciers Move
Glaciers move through processes of plastic deformation and basal slip. As the weight of the ice builds up through accumulation, it begins to exert pressure on the ice, causing movement under the influence of gravity (Video 7.2).
Plastic Deformation At the microscopic scale, individual ice crystals slip past each other as the ice becomes permanently deformed. This motion is analogous to a ball of pizza dough being pressed between the palms. As the ball is pressed, the dough “oozes” outward from the centre of the applied pressure and is permanently deformed. This type of motion allows a glacier to flow a few centimetres per day.
Basal Slip As the pressure increases on the ice at the base of the glacier, partial melting occurs, lubricating the bedrock upon which the glacier flows. This lubrication causes the glacier to move as a single unit or block without plastic deformation. This is like moving the toothpaste by moving the whole toothpaste tube instead of squeezing it. Basal slip allows for faster movement, resulting in glaciers moving several metres per day. Due to higher global temperatures, meltwater can form at the surface of glaciers then be channelled to the base of the glacier through near-vertical tubes called moulins. When meltwater flows along the base of the glacier, it is buoyed and essentially floats on the river of water. This results in the glacier’s moving up to 50 m per day — termed a galloping glacier.
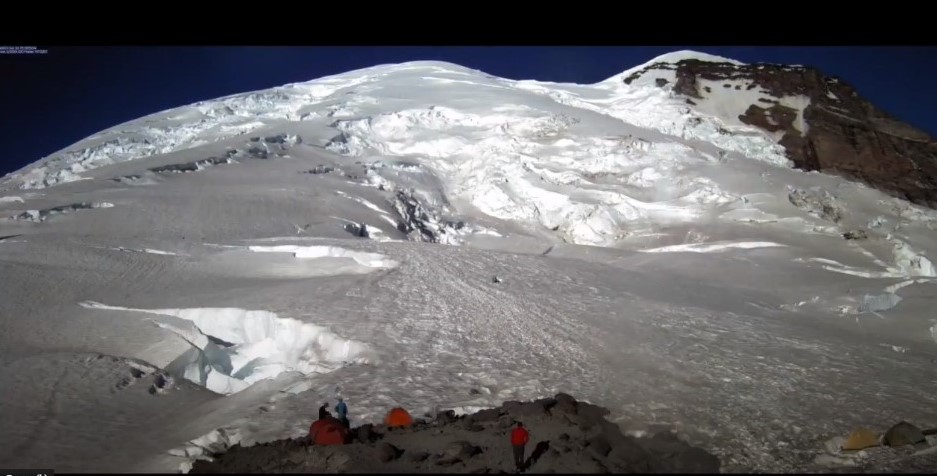
Emmons and Winthrop Glaciers, Mount Rainier National Park, from Wednesday, June 28, 2017 to Monday, September 11, 2017.
Click on the image to load the animation.
Types of Glaciers
By definition, glaciers are large masses of ice on land that are in motion or that show evidence of once having moved. Glaciers can be classified into two groups:
1. Valley Glaciers Also called alpine or mountain glaciers, valley glaciers are “rivers of ice” formed in mountain ranges where snow accumulates, usually in pre-existing river valleys. They flow down these valleys occupying the entire width, and can cover the bedrock under hundreds of metres of ice. However, in warmer climates these glaciers, such as the Furtwangler Glacier on Mount Kilimanjaro in Tanzania (Figure 7.4), occupy only the head regions of a valley on the highest mountain peaks. In high-latitude, colder climates, glaciers may descend many kilometres down the entire length of a valley. In areas where valley glaciers flow down coastal mountain ranges, the end-lobes will terminate at the sea, where the glacier breaks up, forming icebergs (see Figures 7.5 and 7.6).
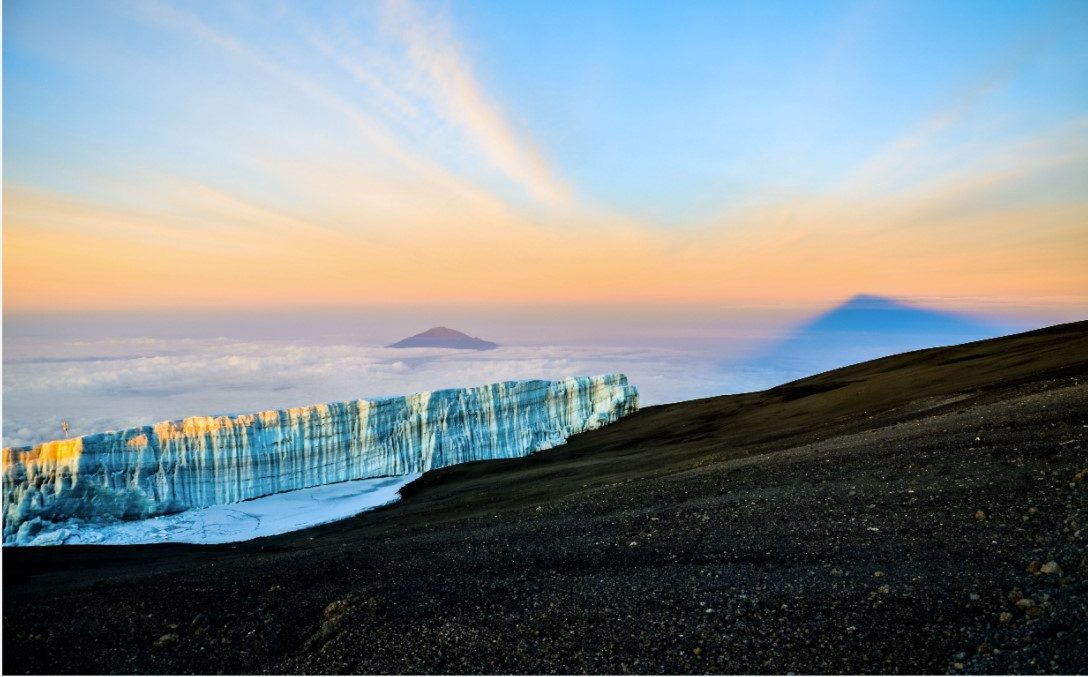
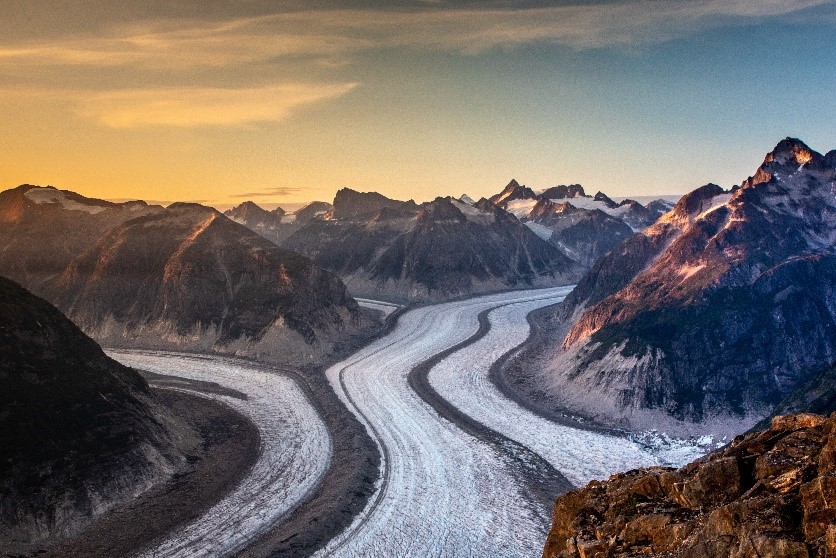
The Colombia Glacier in Alaska is a valley glacier
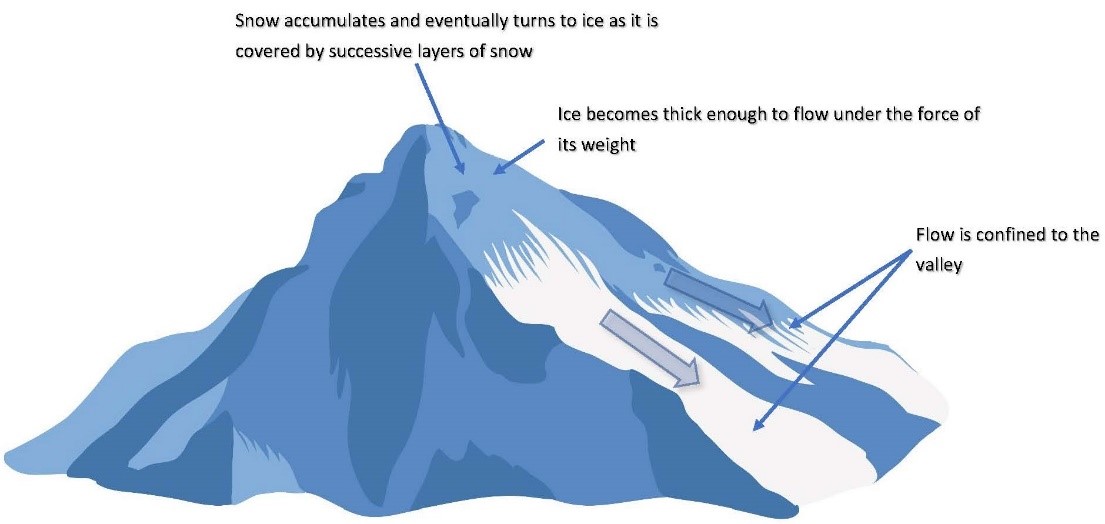
- Continental Glaciers Continental glaciers are very slow moving, thick sheets of ice and are therefore sometimes called ice sheets. They cover a large part of a continent — the largest continental glaciers in existence today cover most of Greenland and Antarctica. Continental glaciers are not confined to a mountain’s valley but essentially cover the entire land surface. Ice caps are the masses of ice formed at Earth’s poles, and include glaciers (Animation 7.1). Most of the Arctic ice cap, located at the north latitudes, lies over water and is not technically considered a glacier. Conversely, almost all of the Antarctic ice cap lies over land — the continent of Antarctica — and is considered a continental glacier.
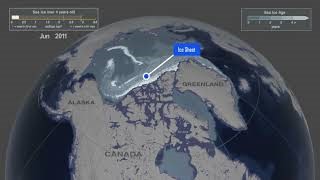
Click on the image to load the animation.
Icebergs
When a glacier meets a large body of water, pieces of ice break off and fall into the water. This process is called calving and produces icebergs (Figure 7.7). The gravitational force that caused the iceberg to calve from the main ice sheet is still incident on the iceberg; however, this force is counterbalanced by the buoyancy created by water that the iceberg displaces. The larger the iceberg, the more water is displaced, increasing the upward buoyancy. Therefore, larger icebergs will seem to ride higher above the water surface than smaller ones.
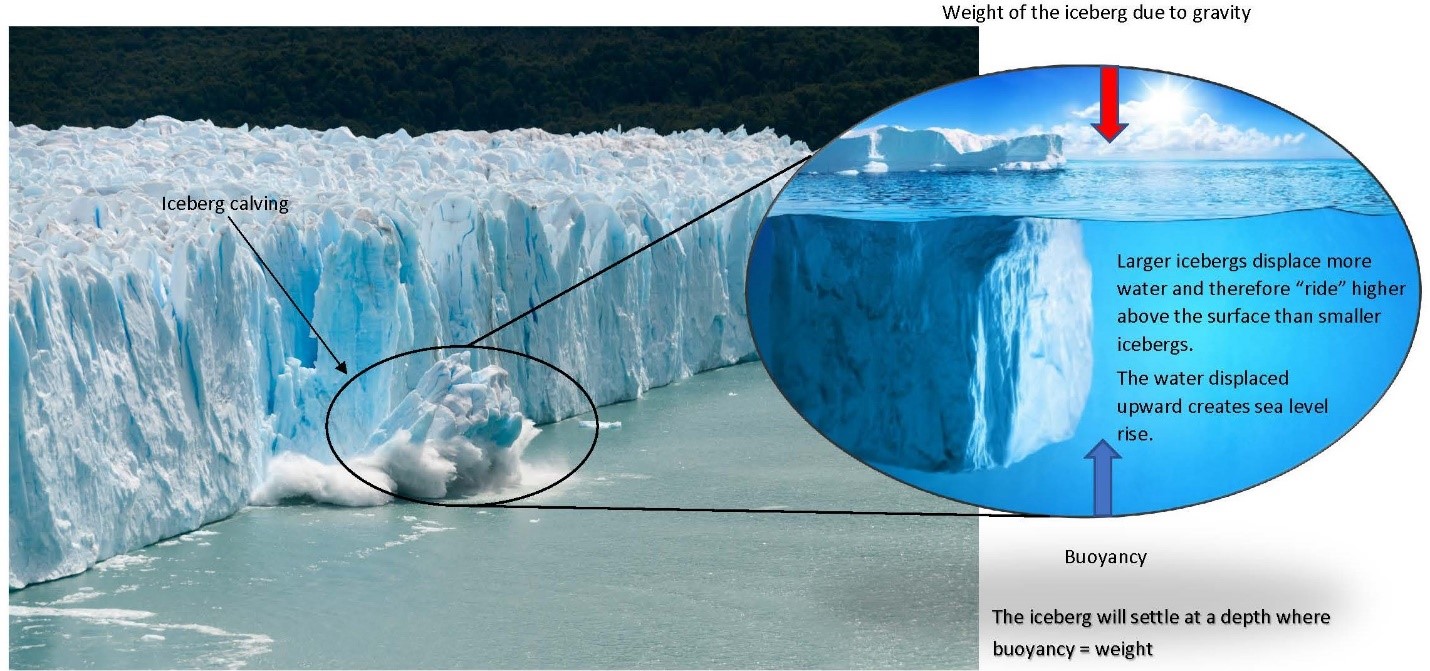
Furthermore, it is this displacement of water that is responsible for rising sea levels — the feared sea-level rise associated with global warming is not just a result of extra water added to the oceans from melting ice but a result of the displaced water from icebergs.
7.2 Glacial Landforms
Just as the impression of your footprint in sand is revealed only after you remove your foot, so too can the erosional effects of a glacier be revealed only after the glacier melts. Only when the ice is removed is its geologic work of erosion and sedimentation revealed. The landforms left behind after glaciation gives an accurate account of the physical processes that shaped them.
Glaciers can erode solid rock and are capable of crushing millions of tonnes of bedrock in a single year. At its base and sides, at the ice–rock boundary, a glacier surrounds fractured and jointed rock, breaking them into pieces and grinding them against the bedrock . The rocks are broken down into a range of sizes, from boulders to fine silt and clay-sized material called rock flour. Rock flour is susceptible to rapid chemical weathering because of its fine size means it has a larger surface area. When melting frees pulverized rock flour from the ice at the edge of the glacier, it dries into dust.
Erosional Landforms
Erosional landforms are landforms created by the scarping and plucking action of glacial ice as it is created and moves downslope. Valley glaciers usually form in existing fluvial systems, widening and deepening the valleys, revealing the new eroded landscape after they have retreated as follows (see also Animation 7.2):
- Striations As a glacier drags rocks along the base, the rocks scratch grooves into the underlying rock pavement. These grooves, called striations (Figure 7.7), provide more evidence of the presence and direction of glacial movement. If you run your hand along the grooves, you will feel how the surface is rough in one direction and smooth in the other. The direction your hand moves when it is smooth is the direction of movement of the glacier when it was flowing.
- Sheep Rocks Advancing glacial ice smoothes out small hills of bedrock known as roches moutonées (“sheep rocks”) (Figure 7.8). The side facing upstream is smooth, and the downstream side is a rough, steep slope due to large pieces of rock having been plucked out by the underside of the moving ice. The contrasting slopes indicating the direction of movement of the glacier that formed the landform.
- Cirques valley glacier carves a series of erosional landforms as it flows from its origin to its lower edge. At the head (beginning) of the glacier, the plucking and tearing action of the flow of ice carves out an amphitheatre-like hollow called a cirque, usually shaped like an inverted frustum of a cone.
- Horns With continued erosion, cirques at the head of adjacent valleys gradually meet at the mountain tops, producing a pointed peak called a horn. As a valley glacier moves down from its cirque, it excavates a new valley or deepens an existing river valley creating the characteristic U-shaped valley (see below). Glacial valley floors are wide and flat with steep walls, in contrast to fluvial valleys, which have V-shaped valleys.
- Arêtes Glaciers flowing in adjacent valleys will eventually widen and meet in sharp, jagged ridges called arêtes along the divide.
- Hanging Valleys Before ice is formed and glaciers flow, the river system upon which the glacial system will be formed has tributaries that will meet the main stream at the same water level. When flowing ice is established in the valleys, the ice flowing from the tributaries to the main glacier will also meet at the same ice level. However, the floor of the tributary valley may be much shallower than the main valley because the ice will deepen the channel. When the ice melts, the remaining tributary valley is left some distance above the main valley, creating a hanging valley (that is, one whose floor lies high above the main floor). The new valley is now occupied by a river, and the junction is marked by a waterfall as the stream in the hanging valley plunges over the steep cliff separating it from the main valley below.
- U-Shaped Valleys As ice in the glacial flow moves through its valley, it widens and deepens the cross-sectional profile. This creates steep-sided valley walls and a wide base, presenting the characteristic U-shaped valley cross-section after the ice has melted.
- Fjords Streams erode their valleys only as low as the baseline (for example, sea level or lake level). Unlike rivers, however, valley glaciers at coastlines may erode their valley floors far deeper than sea level. When the ice retreats, the steep-walled valleys (with the U-shaped profile) are flooded with seawater. These flooded post-glacial valleys are called fjords.
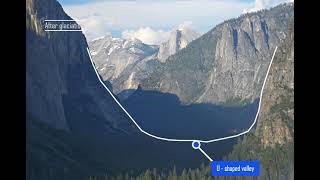
Click on the image to load the animation.
Depositional Landforms
Glaciers transport eroded rock material of all kinds and sizes downstream, eventually depositing them where the ice melts. Because debris picked up by ice cannot settle out, it is transported far. Like water and wind currents, flowing ice has a high transport capacity to carry particles of a certain size. That is, ice has extremely high competence, which is the largest particle size a glacier can carry (ice can carry huge blocks many metres in size that no other transporting agent could move). Ice’s tremendous capacity can also be seen visually: some ice is so full of rock material that it appears to have a dark colour.
When glacial ice melts, it deposits a poorly sorted (or well-graded) heterogeneous load of boulders, cobbles, pebbles, sand, and clay (that is, a wide range of grain sizes). This wide range of particle sizes is the characteristic that differentiates glacial sediment from fluvial or aeolian (much better sorted) sediments. The term drift is used for all material of glacial origin found anywhere on land or sea.
Some drift is deposited directly by melting ice. This unstratified and poorly sorted sediment is known as till, and it may contain all sizes of fragments, including the large boulders called erratics. These are the boulders you can see collected in farmers’ fields across southern Ontario. Other deposits of drift are laid down by meltwater streams flowing in tunnels within and beneath the ice and in streams at the ice fronts. Like any other water-born sediment, this material is stratified, is well sorted, and may be cross-bedded due to ripple/dune migration. Drift that has been carried, modified, sorted, and distributed by meltwater streams is called outwash.
Ice-Laid Deposits
Ice-laid deposits are deposits of till left directly by the retreating ice in various forms:
- Moraines Moraines are accumulations of coarse rock, sand, and clay material carried by ice or deposited as till. There are many types of moraines, named for their position with respect to the glacier that formed it (Figure 7.9). The most prominent in size and appearance is the end moraine, formed at the ice front. The end moraine at the farthest point the ice front reaches is called the terminal moraine. As ice flows downslope, it brings more and more sediment to its melting edge. The unsorted material accumulates there as a hilly ridge of till perpendicular to the flow. When this unsorted sedimentary material is deposited at the side of the glacier, parallel to the flow, it is termed a lateral moraine. The lateral moraines of adjacent glaciers also merge when the glaciers merge, creating a medial moraine. Unsorted sediment deposited at the base of the glacier is termed a ground moraine.
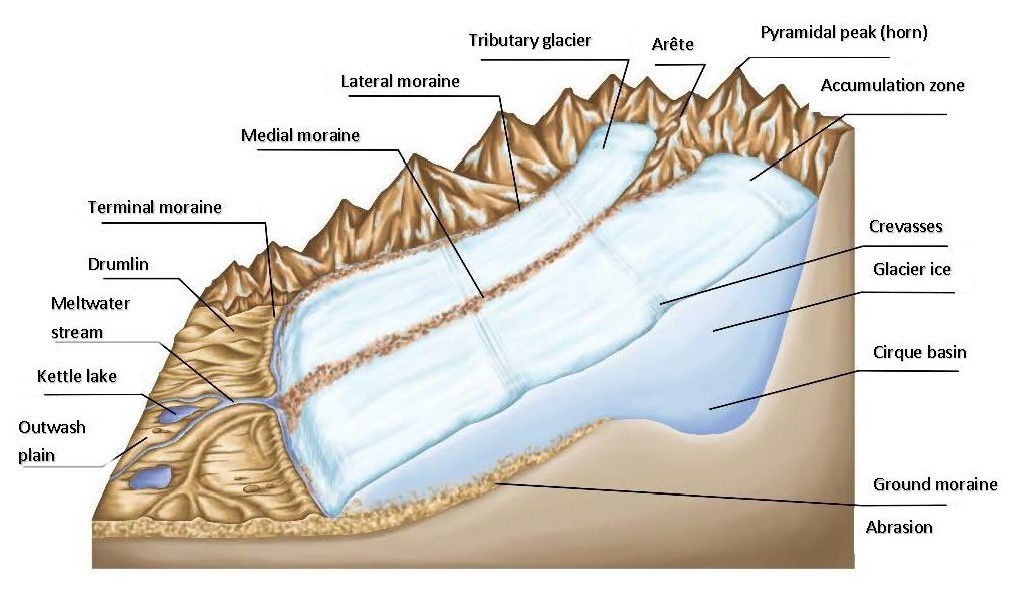
- Drumlins Some continental glacial terrains, unconstrained by valleys, display prominent landforms called drumlins. These are large, streamlined hills of till and bedrock that parallel the direction of ice movement. Drumlins are usually found in clusters and shaped like long, inverted spoons, or submerged eggs with the steep slope pointing upstream and gentle slopes pointing downstream (Figure 7.10 and Video 7.3). They may be 25–50 m high and 1 km long).
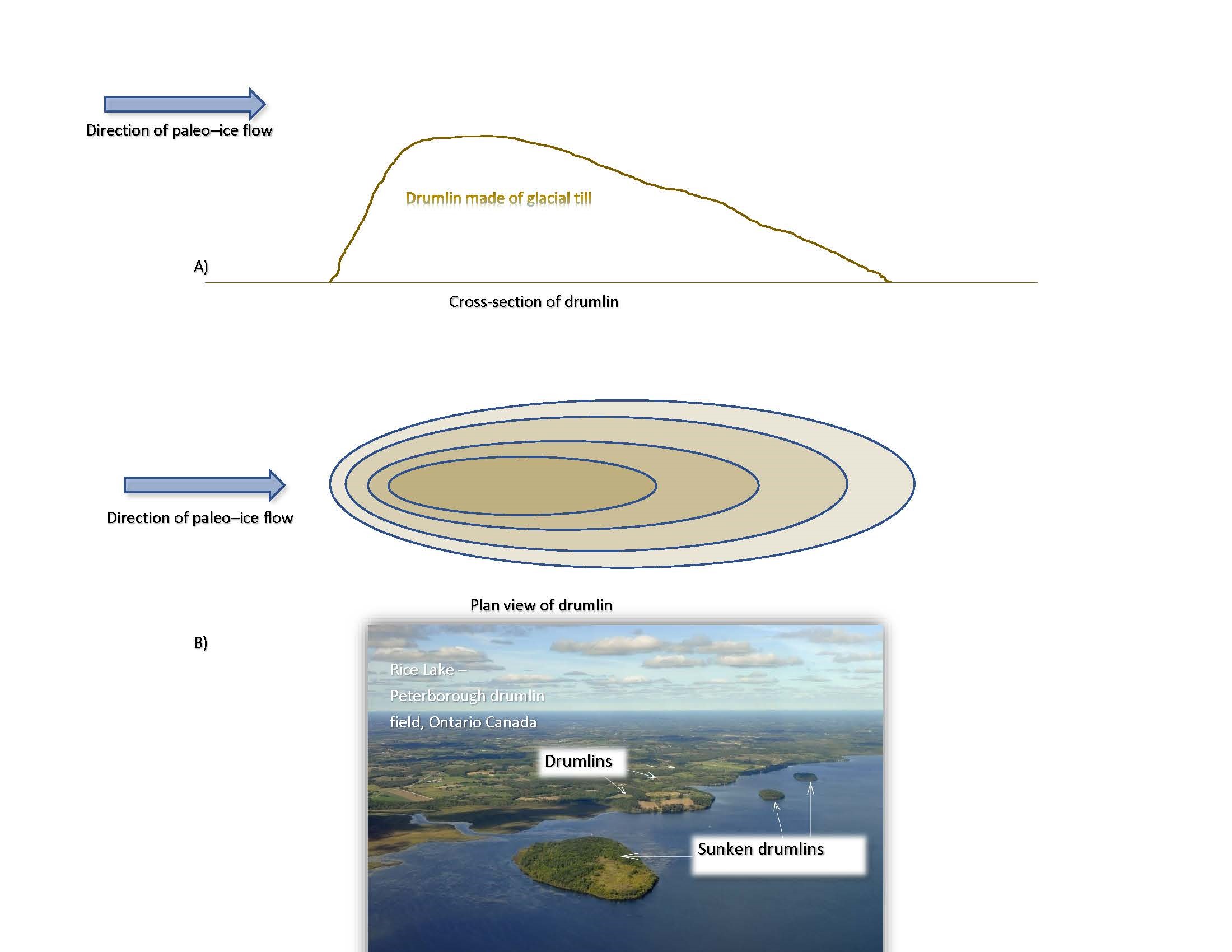
(a) Cross-section indicating direction of paleo ice flow. (b) Drumlin field near Peterborough, Ontario
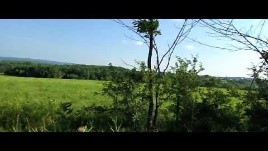
Click on the image to load the video.
Water-Laid Deposits
Water-laid deposits are deposits from outwash from glacial meltwaters that take a variety of forms (see also Figure 7.11):
- Kames Kames are small hills of sand and gravel deposited at or near the edge of the ice. Some kames are deltas built into lakes at the ice edge. When the lakes drain, the deltas are preserved as flat-topped hills and are often exploited as commercial sand and gravel pits.
- Kettles Kettles, which are predominant in the Oak Ridges Moraine, are formed when pieces of ice fall off the ice front as the continental glacier retreats. These pieces of ice are subsequently covered by meltwater sediments that create a steep-sided depression when the ice melts. The depression may be occupied by water, if it intersects the water table, creating ponds or lakes called kettle lakes. Meltwater from the ice front may deposit silt and clays at the bottom of a kettle lake in a series of alternating coarse and fine layers called varves. A varve is a pair of layers formed in one year by seasonal freezing of the lake surface. In the spring and summer, when meltwater is more energetic, coarse silts and fine clays find their way into the lake. During the winter, when the lake freezes over, the coarse sediments settle first, followed by the finer clays, creating the varve pair.
- Eskers eskers are long, narrow, winding ridges of sand and gravel found in the middle of ground moraines. They can be of the order of kilometres long in a direction roughly parallel to the direction of ice movement. The origin of eskers is suggested by the well sorted, water laid character of the materials and the sinuous, channel-like course of the ridge. Eskers were deposited by melt water streams flowing in tubes along the bottom of the melting glacier. The tubes themselves were open by water seeping through crevasses and cracks in the ice. Eskers can be recognized in aerial photographs (plan view) by their resemblance to stream morphology but with sediments sticking out on top of the landscape where the river valley would be. Video 7.4 shows the locations of some Central Ontario eskers.
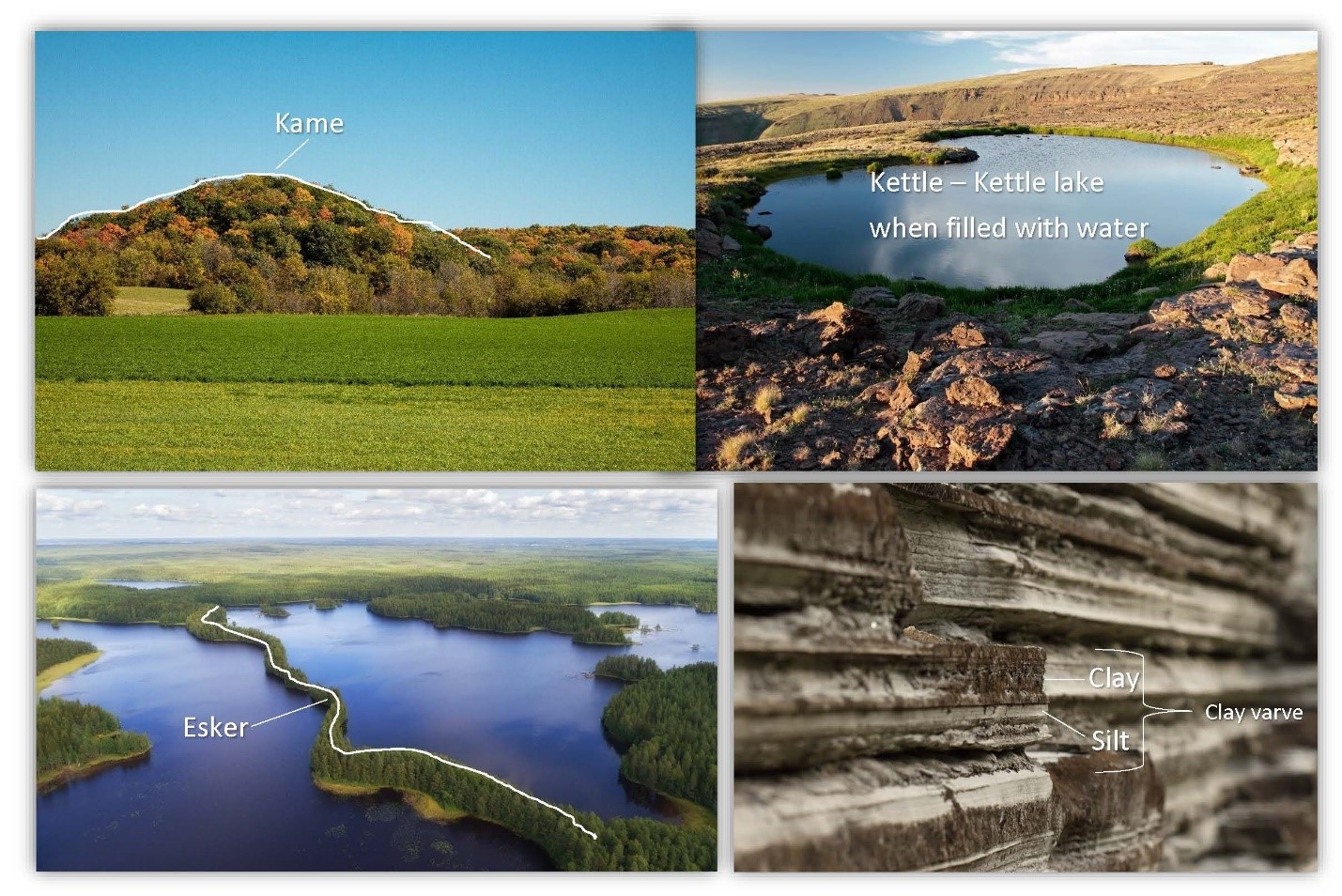
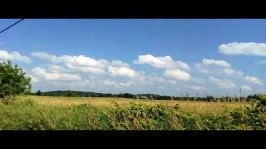
Click on the image to load the video.
7.3 The Oak Ridges Moraine
The Oak Ridges Moraine is one of the largest remnants of the last glaciation in Southern Ontario, stretching 160 km from the Niagara Escarpment in the west to east of Peterborough beyond Rice Lake (Figure 7.12). Some places along this ridge can be as much as 20 km wide, displaying a hummocky surficial topography due to the presence of kettles. The Oak Ridges Moraine was formed between two glacial lobes about 13,000 years ago during the last glaciation.
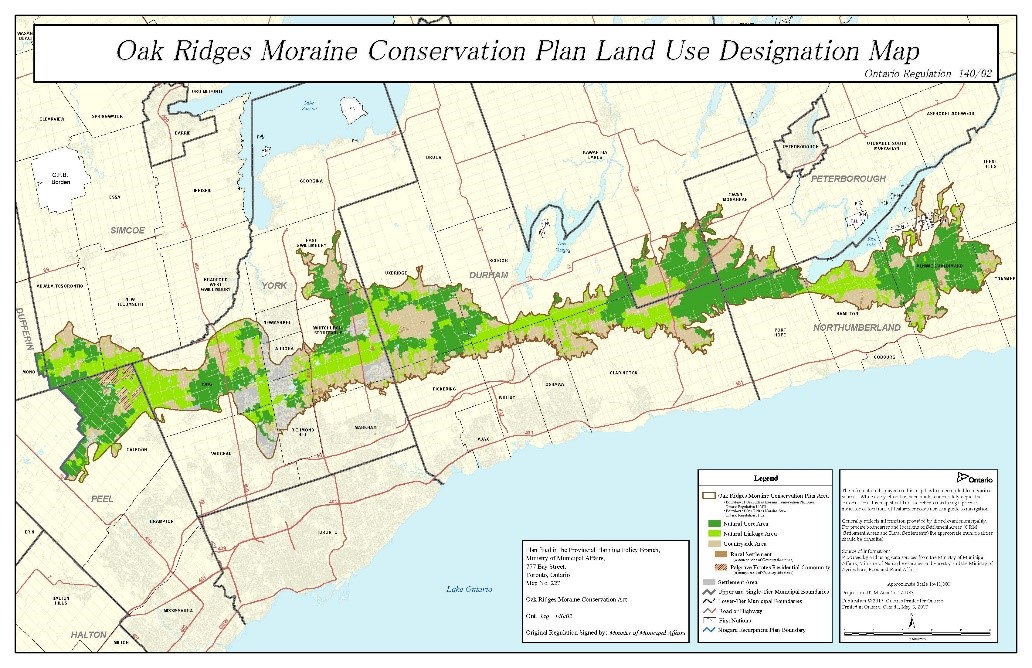
The moraine was formed when sediments were poured into a lake trapped between the Simcoe and Ontario Lobes — two continental glacial lobes — and the Niagara Escarpment (Figure 7.13). Meltwaters from the glacial lobes deposited huge amounts of sand and gravel as deltas in the lake, eventually building up enough sediment to cover the margins of the two lobes. After the end of the glaciation, the large pieces of ice left behind by the retreating glacial lobes melted under the sediments, creating the many kettle lakes associated with the Oak Ridges Moraine today.
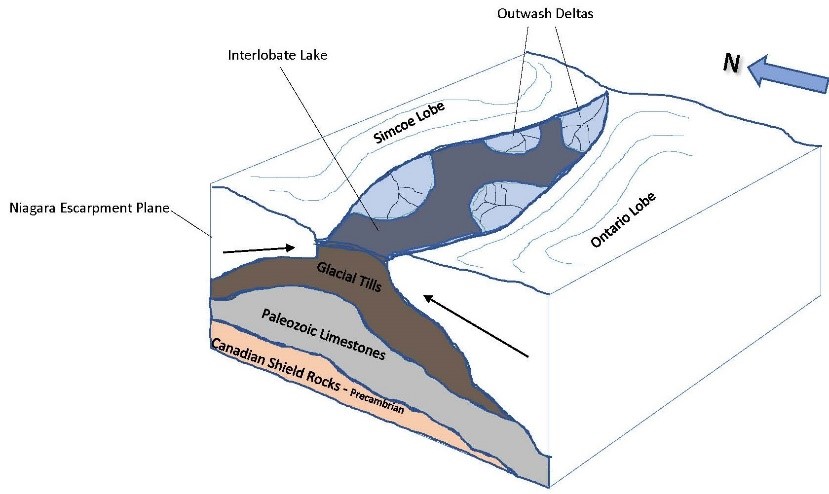
The moraine formed between lobes of the Laurentide Ice Sheet and the Niagara Escarpment about 13,000 years ago during the final stages of the last glaciation.
Granular ice with low air content
For glaciers, the amount of snow and ice added in one year
For glaciers, the amount of snow and ice lost in one year
For glaciers, glacial motion by of individual ice crystals’ passing each other
For glaciers, glacial motion as a block over lubricated bedrock
A near-vertical tube that forms in a glacier
A glacier that moves at up to 50 metres per day
Large masses of ice on land that are in motion or that show evidence of once having moved
River-like glaciers formed in mountain ranges
Slow-moving, thick sheets of ice covering a large part of a continent
Polar ice masses
Finely powdered rock material produced by weathering that occurs during glacial erosion
Landforms created by the scarping and plucking action of glacial ice as it is created and moves downslope
Grooves in rock created by glaciers dragging rock over it
Small glacier-created bedrock hills; also called “sheep rocks”
A roughly circular basin with cone-shaped sides
A pointed peak of a mountain created by adjacent cirques
Ridges where adjacent glacial valleys meet
A tributary valley with a floor above that of the valley its waters empty into
A glacier-formed valley with steep sides and a wide base
Flooded post-glacial steep U-shaped valleys
For ice, the quantity of particles of rock that it can carry
The measure of the largest size of rock particle a stream or glacier can carry
Material of glacial origin
Unstratified, poorly sorted glacial deposit]
Large boulders deposited by glaciers
Drift that has been carried, modified, sorted, and distributed by meltwater streams
Deposits of till left directly by retreating glaciers
Accumulations of coarse rock, sand, and clay material carried by ice or deposited as till
Prominent glacial deposit at the ice front
Glacial deposit at the farthest point reached by an ice front
Glacial sedimentary deposits parallel to glacier flow
Merged lateral moraines from adjacent glaciers
Unsorted sediment deposited at the base of the glacier
Large, streamlined hills of till and bedrock
Glacial meltwater outwash deposits
Small hills of sand and gravel deposited at or near the edge of a glacier
Depressions formed when ice left by glaciers melts beneath sediments
Kettles occupied by water
A pair of sediment layers formed by one year’s seasonal lake-surface freezing and thawing
A ridge of sand and gravel in a ground moraine